FUTURE ENERGY eNEWS
Integrity Research Institute DECEMBER 2014
|
|
|
|
END OF YEAR FUNDRAISING
DRIVE
or
Make a Tax deductible gift
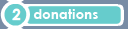
THANKS FOR YOUR
GENEROSITY!
IRI is a 501(c)(3)
charitable organization dedicated to educating the public on
eco-friendly emerging energy technologies.
Your gift is
tax-deductible to the full extent provided by law.
|
HAPPY HOLIDAYS
FROM ALL AT
LIKE US ON FACEBOOK

AND
GET 10% OFF ANY ITEM ON OUR CATALOG
|
|
|
Dear Subscriber, HAPPY HOLIDAYS!
|
This month IRI is engaged in its once-a-year Fund-Raising
Campaign. We depend upon you for continuing the IRI all-volunteer
effort to help the world with emerging energy, propulsion and
bioenergetics information and we have no payroll expenses for a
nonprofit organization, so your donation goes much farther. You can help
us make a difference in the academic, commercial, and private arenas
with your tax-deductible donation (#2 button on left)
ormembership (#1 button). If you become an
IRI Member before December 31, 2014, we
will send directly to you this year's annual Member's gift and next
year's too -- annual gifts for TWO years in a
row! You might also consider getting that last minute Holiday gift such
as the popular EM
Pulser with a 30-day money back guarantee and one-year
warranty.
As IRI Members know, we align ourselves with the Union of
Concerned Scientists (UCS) in many of their scientific
and energy areas and often send copies of the UCS Catalyst magazine in
our quarterly mailings to Members. Well, this month Four
Champions of Science are up for a vote and all of them are
inspiring. We and UCS applaud their efforts and want to recognize them
for what they've accomplished. We also want to give you
the chance to vote for the one whose story most inspires you.
Another surprise this month
was receiving an invitation from The International Journal of
Geosciences (IJG, ISSN Online: 2156-8367), a peer-reviewed
open-access journal, seeking papers for the upcoming special issue on "Gravity Research". We would like to
invite you to submit or recommend original research papers to this
issue through ourPaper Submission System. (Submission
Deadline is December 18, 2014).
Our Story #1 gives us hope that inertial and magnetic confinement
fusion might still possibly be viable forms of fusion, with Lockheed
Martin and companies like Fusion Power, General Fusion, and Helion in
the game. The story also mentions one of the IRI affinity company
Lawrencevile Plasma Physics, which has used crowdfunding to
further advance proton-boron fusion, an alternative and perhaps the
most simple and exciting form of fusion today since it is four times a
powerful as hitting two deuterons together. See www.futurenergy.org for
a summary of CEO Eric Lerner's presentation at the IRI COFE3 event and
also our Videos for his brief trailer.
Story #2 reveals an amazing discovery that in 2013 it was found
that infrared laser pulses could increase the quantum coupling and make
the ordinary cold superconductor YBCO transform
at room temperature and start superconducting for a
few picoseconds with NO cooling at all. This give great hope for
solving the room temperature problem for all superconductors in the
near future. Why do we need superconductors? Well, Story #3 tells the
intriguing and surprising tale of high voltage cables
connecting Norway to several countries which are perfect for
superconductors when they become operable at room temperature. Norway
has figured out the simplest energy storage game in the world: pump
water. When they can reduce the electrical power cost to 6 to 7
cents per kWh instead of 9 to 12 cents per kWh, then the rest of Europe
wants to connect to Norway for gigawatts of power without damaging the
environment.
Story #4 gives us a great overview for the good news about the
spike in the Solar Power Revolution. The US is now reported to
have about 13 GW of solar power installed to power 2.4 million
households. To continue the growth spurt, important focus areas are
cited including renewable electricity standards for every state and
solar tax credits if our new Congress will stop thinking about the
Keystone fossil fuel pipe and instead, look toward saving the
environment with long-term solutions that our decendants will thank us
for.
Talking about decendants, there is no doubt that some of them
will live on Mars for one reason or another. How about if it were
possible, as Story #5 tells us, to split water into oxygen and hydrogen
but save the mix for release with a simple platinum catalyst? That is
what the University of Glasgow
has
accomplished. In return, it is estimated 30 times as much
hydrogen can be made from the process than with existing
systems
for the same power input, since only a single pulse of energy is
needed.
Have a Happy
Holiday and Prosperous New Year!
|
|
|
|
1) THE DYNOMAK, A FUSION
TECHNOLOGY CHEAPER THAN COAL
|
By
Evan Ackerman IEEE SPECTRUM
DECEMBER 2014
http://spectrum.ieee.org/energy/nuclear/inside-the-dynomak-a-fusion-technology-cheaper-than-coal
Modifying the most common type of experimental
reactor might finally make fusion power feasible

Fusion Power has
many compelling arguments in its favor. It doesn't produce dangerous,
long-term toxic waste, like nuclear fission. It's far cleaner than coal,
with a supply of fuel that's virtually unlimited. And unlike with wind
and solar, the output of a fusion power plant would be constant and
reliable.
The primary argument
against fusion power has been that despite decades of work, it still
doesn't exist. But that's no hindrance to a fresh crop of enthusiasts
from academia, government, private industry, and even venture capital
firms.
In October, Lockheed
Martin Corp. revealed that it's been working on a type of fusion reactor
that could be made small enough to transport by truck.Lawrenceville Plasma Physics raised money
through crowdfunding in June to advance its alternative
proton-boron fusion. Helion Energy is developing a type of fusion
based on magnetic compression, and General Fusion is working toward a
power system that involves shock waves inside a vortex of liquid metal.
A particularly promising
approach was unveiled recently by a University of Washington research
group, led byplasma physicist Tom Jarboe. They've been
developing a type of fusion reactor called a dynomak. The researchers
involved say the technology is unique in that it offers a path to a power
plant that's backed up by demonstrated physics and because such a reactor
also promises to be even more economical than a coal-fired power plant.
The dynomak is a
variation of the most popular type of research fusion machine, the tokamak. Essentially, a tokamak is a
doughnut-shaped machine that generates helical magnetic fields by
combining toroidal fields (which go around the doughnut's equator) with
poloidal fields (which wrap around the outside of the doughnut). These
fields have to be strong enough to keep plasma stable and contained
indefinitely at the tens to hundreds of millions of degrees Celsius
necessary to induce fusion.
In practice, tokamaks are
hollow, doughnut-shaped vacuum chambers with interior walls made of
heat-resistant metals or ceramics. Outside the chamber are massive
superconducting coils that generate the toroidal magnetic fields that
stabilize the plasma. The European Union, China, India, Japan, Russia,
South Korea, and the United States are collaborating to build a giant US
$50 billion tokamak in France called ITER (originally International
Thermonuclear Experimental Reactor), which may lead to a fusion power
plant in the 2030s. But the University of Washington group-and its
alternative-fusion competitors-are hoping to beat it to
commercialization.
The University of
Washington's dynomak is a refinement of a subtype of tokamak called a
spheromak. The most important difference is that the spheromak does away
with most of the tokamak's expensive superconducting magnetic coils.
Instead, a spheromak uses the electric currents flowing though the plasma
itself to generate the magnetic fields needed to both stabilize and confine
the plasma.
This is tricky, as UW
graduate student Derek Sutherland explains. For it to
work, you need not only a sophisticated understanding of the physics
underlying the behavior of the plasma but also a very efficient way of
driving the current. If you're not careful, you'll end up dumping all the
energy that your reactor is producing right back into the plasma just to
keep it contained-resulting in a very expensive machine that will power
itself and nothing else.
According to Sutherland,
the big breakthrough was UW's experimental discovery in 2012 of a
physical mechanism called imposed-dynamo current drive (hence
"dynomak"). By injecting current directly into the plasma,
imposed-dynamo current drive lets the system control the helical fields
that keep the plasma confined. The result is that you can reach
steady-state fusion in a relatively small and inexpensive reactor.
"We are able to drive plasma current more efficiently than
previously possible," says Sutherland. "With that efficiency
can come higher current and a more compact, economical design."
How economical? According
to projections by Sutherland's group, a dynomak has the potential to cost
less than a tenth as much to build as a tokamak like ITER, even as it
produces five times as much power. This massive boost in efficiency is
very compelling: According to UW's analysis, it makes the total cost of a
dynomak fusion power plant with an output of 1 gigawatt slightly cheaper
than the total cost of a coal power plant with the same output-$2.7
billion versus $2.8 billion.
The UW researchers are
particularly optimistic about their dynomak because it's not much of a
deviation from established systems. "I think we've blended the
mainstream and alternates into a pathway that is completely plausible but
different enough to really start addressing the economic issues facing
fusion power," says Sutherland.
"The spheromak-and
the dynomak is a species of spheromak-in particular has not received the
level of attention that it warrants," says University of Iowa
physicist Fred Skiff. "The potential advantages
are significant: a lower magnetic field-and therefore lower cost and
complexity-and a smaller reactor." The lower magnetic field
requirements are important because "large superconducting coils are
not trivial to produce and protect in a reactor environment."
However, "there are
significant unknowns," says Skiff. "The ability to control the
current profile, the plasma position, and the ability to maintain high
confinement will have to be demonstrated."
The next steps for the
dynomak are straightforward. The experimental device Jarboe's group is
working with right now, called HIT-SI3, is about one-tenth the size that
a commercial dynomak fusion reactor would be. It includes three helicity
injectors, which are the coils that control the delivery of twisting
magnetic fields into the plasma. "The eventual dynomak reactor will
have six injectors according to the current design," says
Sutherland. With $8 million to $10 million in funding, the group hopes to
construct HIT-SIX, a six-injector machine that will be twice as large as
HIT-SI3.
At that size, things
start to get interesting, says Sutherland. HIT-SIX is designed to reach
millions of degrees Celsius using a mega-ampere of plasma current. If
imposed-dynamo current drive works well in HIT-SIX, he'll be "much
more confident going forward that our development path will be
successful," he says.
That entire path,
including an electricity-generating pilot plant, would require about $4
billion, Jarboe's group projects. Compared with ITER's $50 billion,
that's a bargain.
FUSION
ALTERNATIVES
LAWRENCEVILLE PLASMA PHYSICS
Funding: $3 million
from private investors, plus $180,000 from a crowdfunding campaign on
Indiegogo; currently applying for a two-year, $2 million Advanced
Research Projects Agency-Energy grant. How does it work? A
strong pulse of electricity generates filaments of plasma. The filaments
are combined, and natural instabilities cause them to twist into a
plasmoid. The plasmoid self-heats to reach a fusion state. What's
the advantage? Instead of trying to control plasma instabilities
with magnetic fields, the system uses the instabilities to create fusion.
Hydrogen-boron fusion reactions do not create radioactive
by-products. When will it be commercial? Currently performing
test shots to increase plasma density; garage-size 5-MW generators by 2020
costing $300,000 to $500,000 each.
LOCKHEED
MARTIN :
Funding: Internal. How
does it work? The device [above] is similar to a tokamak, but it
uses a new type of self-tuning feedback mechanism to control its magnetic
field geometry. What's the advantage? Very efficient reactors
will be small enough to fit inside trucks and shipping containers, and
they could even power an airplane indefinitely.When will it be
commercial? Currently testing operational theories; functional
reactor by about 2025.
HELION ENERGY:
Funding: US $7 million from NASA, the Department of
Energy, and the Department of Defense, plus $1.5 million in seed
funding.How does it work? Plasma fuel forms stable toroids at either
end of a chamber. The toroids are then slammed together at more than1.6
million kilometers per hour. What's the advantage? All
solid-state electronics make for small, modular power plants. Fusion
energy is directly converted to electricity. The plant generates its own
helium-3 fuel as a by-product. When will it be commercial?Currently
developing a reactor-scale fusion core; 50-megawatt pilot plant in 2019.
GENERAL FUSION:
Funding: $55
million, primarily venture capital.How does it work? Magnetic fields
briefly confine plasma inside a vortex of liquid metal. Steam-
pistons create a spherical shock wave, which collapses the liquid metal
vortex, compressing the fuel to achieve a burst of fusion
energy. What's the advantage?Plasma needs only a brief confinement,
and steam-powered pistons lead to a simple, cheap reactor. When will
it be commercial?Currently testing reactor subcomponents; successful
prototype in 2015 could lead to commercial reactor in 2020.
back to table of contents
|
2)
Superconductivity without Cooling
|
Phys.Org, December
4th, 2014 in Physics / Superconductivity
No resistance at room
temperature: The resonant excitation of oxygen oscillations (blurred)
between CuO2 double layers (light blue, Cu yellowy orange, O red) with
short light pulses leads to the atoms in the crystal lattice briefly
shifting away from their equilibrium positions. This shift brings about
an increase in the separations of CuO2 layers within a double layer and a
simultaneous decrease in the separations between double layers. It is
highly probable that this enhances the superconductivity. Credit: Jörg
Harms/MPI for the Structure and Dynamics of Matter.
Superconductivity is a
remarkable phenomenon: superconductors can transport electric current
without any resistance and thus without any losses whatsoever. It is
already in use in some niche areas, for example as magnets for nuclear
spin tomography or particle accelerators. However, the materials must be
cooled to very low temperatures for this purpose. But during the past
year, an experiment has provided some surprises.
With the aid of short
infrared laser pulses, researchers have succeeded for the first time in
making a ceramic superconducting at room temperature - albeit for only a
few millionths of a microsecond. An international team, in which
physicists from the Max Planck Institute for the Structure and Dynamics
of Matter in Hamburg have made crucial contributions, has now been able
to present a possible explanation of the effect in the
journal Nature: The scientists believe that laser pulses cause
individual atoms in the crystal lattice to shift briefly and thus enhance
the superconductivity. The findings could assist in the development of
materials which become superconducting at significantly higher
temperatures and would thus be of interest for new applications.
In the beginning,
superconductivity was known only in a few metals at temperatures just
above absolute zero at minus 273 degrees Celsius. Then, in the 1980s,
physicists discovered a new class, based on ceramic materials. These
already conduct electricity at temperatures of around minus 200 degrees
Celsius without losses, and were therefore called high-temperature superconductors. One of
these ceramics is the compound yttrium barium copper oxide (YBCO). It is
one of the most promising materials for technical applications such as
superconducting cables, motors and generators.
The YBCO crystal has a
special structure: thin double layers of copper oxide alternate with thicker
intermediate layers which contain barium as well as copper and oxygen.
The superconductivity has its origins in the thin double layers of copper
dioxide. This is where electrons can join up to form so-called Cooper
pairs. These pairs can "tunnel" between the different layers,
meaning they can pass through these layers like ghosts can pass through
walls, figuratively speaking - a typical quantum effect. The crystal only
becomes superconducting below a "critical temperature",
however, as only then do the Cooper pairs tunnel not only within the double
layers, but also "spirit" through the thicker layers to the
next double layer. Above the critical temperature, this coupling
between the double layers is missing, and the material becomes a poorly
conducting metal.
The result helps material
scientists to develop new superconductors
In 2013, an international
team working with Max Planck researcher Andrea Cavalleri discovered that
when YBCO is irradiated with infrared laser pulses it briefly
becomes superconducting at room temperature. The laser light had
apparently modified the coupling between the double layers in the
crystal. The precise mechanism remained unclear, however - until the
physicists were able to solve the mystery with an experiment at the LCLS
in the US, the world's most powerful X-ray laser. "We started by
again sending an infrared pulse into the crystal, and this excited
certain atoms to oscillate," explains Max Planck physicist Roman
Mankowsky, lead author of the current Nature study. "A short time
later, we followed it with a short X-ray pulse in order to measure the
precise crystal structure of the excited crystal.
The result: The infrared
pulse had not only excited the atoms to oscillate, but had also shifted
their position in the crystal as well. This briefly made the copper
dioxide double layers thicker - by two picometres, or one hundredth of an
atomic diameter - and the layer between them became thinner by the same
amount. This in turn increased the quantum coupling between the double
layers to such an extent that the crystal became superconducting at room
temperature for a few picoseconds.
On the one hand, the new
result helps to refine the still incomplete theory of high-temperature
superconductors. "On the other, it could assist materials scientists
to develop new superconductors with higher critical temperatures,"
says Mankowsky. "And ultimately to reach the dream of a
superconductor that operates at room temperature and needs no cooling at
all." Until now, superconducting magnets, motors and cables must be
cooled to temperatures far below zero with liquid nitrogen or helium. If
this complex cooling were no longer necessary, it would mean a breakthrough
for this technology.
More
information: "Nonlinear lattice dynamics as a basis for
enhanced superconductivity in YBa2Cu3O6.5." Nature 516 71, DOI: 10.1038/nature13875
Provided by Max Planck
Society
Commercialization and
plans to continue developing and scaling up its prototypes
|
3)
Norway Wants to be Europe's Battery
|
By Peter Fairley
IEEE SPECTRUM
The Viking
Connection: A new high-voltage DC cable will connect Denmark to
Norway.

Norway's
hydropower reservoirs make up nearly half of Europe's energy storage
capacity. European grid operators need energy storage to cope with an
ever-mounting, always-shifting torrent of wind power. See the connection?
So does Norway. In December, engineers will energize a new subsea power
cable that will begin to bridge the gap between need and opportunity,
greatly expanding European power systems' access to Norway's
hydropower-rich power grid.
The
240-kilometer cable across the Skagerrak Strait separating southern
Norway and northern Denmark is Norway's first new power link to Denmark
since 1993. Called Skagerrak 4, its high-voltage direct current (HVDC)
converters-the electronic units at either end of the line that transform
AC into high-voltage DC and vice versa-are also the building blocks for
more ambitious cables from Norway to wind-power heavyweights Germany and
the United Kingdom. Construction on those is expected to commence during
the coming year.
The
existing Skagerrak interconnection, three HVDC cables with a combined
1,000 megawatts of capacity, is already showing the world just how well
wind and hydropower complement each other. According to the Danish Energy
Agency, such interconnectors are why Denmark can accommodate the world's
highest levels of wind power, which met 41.2 percent of Danish demand in
the first half of this year. At times wind power production even exceeds
the country's domestic power demand.
"We
store their surplus in the hydro reservoirs and then feed it back on a
seasonal basis or a daily basis. This is a very strong business
case," says Håkon Borgen, executive vice president at Statnett,
Norway's state grid operator.
Norwegian
hydropower turbines throttle down as Norway consumes Danish wind energy
instead, leaving an equivalent amount of energy parked behind dams. And
when the weather shifts and becalms the North Sea winds, the reservoirs
and Skagerrak's cables feed that stored energy back to Denmark.
Borgen
says the addition of the 700-MW Skagerrak 4 advances plans to plug the
U.K. and Germany into Norway's batteries by pushing the most flexible
form of HVDC technology-voltage source converters (VSCs)-to its highest
voltage yet. VSCs' ability to stabilize the voltage of the AC grids on
both sides of a cable makes the technology better suited than any
existing alternative for handling intermittent flows of renewable energy,
he says. Skagerrak 4's VSCs operate at 500 kilovolts each-30 percent
higher than the previous record holder. Borgen says that voltage boost
will be needed to reduce losses on longer runs such as the 720-km cables
to the U.K., which will be the world's longest subsea power cables.
ABB,
of Zurich, which built Skagerrak 4's VSCs, says the tougher technology
challenge was ensuring that the VSCs function well alongside the older
HVDC lines. That's because current that Skagerrak 4 carries south across
the strait must cycle back to Norway via the Skagerrak 3 cable, which
uses the older, classic HVDC converters. This will be the world's first
paired operation of cables using VSCs and classic HVDC converters.
Such
a pairing gets interesting when operators want to reverse the flow of
power-something that can happen up to 1,000 times per year at Skagerrak
as winds and markets shift. VSCs normally reverse power flow by reversing
a line's current, whereas classic HVDC converters must flip the line's
voltage polarity.
So
how to reverse power flow on both lines? ABB's solution is a 5- to
10-second process that uses coordinated actions by both converter types
and eight high-speed switches that reconfigure the wiring of the VSCs,
flipping their polarity so that the flow of power in Skagerrak 4 can
change direction while its current keeps flowing south.
The
process interrupts the circuit by which current flows from one cable to
the other. But Lars-Erik Juhlin, an HVDC expert at ABB, says there is no
meaningful loss or surge in power to the AC grids.
The
key, explains Juhlin, is the excellent electrical conductivity of
seawater. When the power-reversal scheme interrupts the circuit, the
converters use subsea electrodes at either shore to feed the return
currents across the strait through the water. Sending current through
seawater can corrode subsea infrastructure such as natural gas pipelines,
but here, the dose makes the poison. "They can accept even 2,000
amps for up to 2 hours. So for a short pulse, it's no problem," says
Juhlin.
Statnett's
follow-on interconnection projects could move quickly because they will
just be longer versions of Skagerrak 4. The first, a pair of 500-kV VSC
cables between Norway and Germany, cleared final regulatory approvals
last week. Statnett and its European grid partner, Dutch-German firm
Tennet, foresee charging up the 1,400-MW NordLink in 2018. The pair of
Norway-U.K. cables, a joint effort of Statnett and London-based National
Grid, is slated to start by 2020.
There
should be many more cables to come if European countries make good on
official goals to eliminate carbon emissions from power generation by
2050. The German government's Advisory Council on the Environment, for
example, concluded in its influential 2011 report that an optimal
zero-carbon power system for Germany would need more than 40 gigawatts of
interconnection to Norway. That system, the council projected, would
deliver power at a very affordable 6 to 7 euro cents per kilowatt-hour.
Without Norwegian storage, power costs would rise to 9 to 12 euro cents
per kilowatt-hour.
Ånund
Killingtveit, a professor of hydraulic and environmental engineering at
the Norwegian University of Science and Technology, says Norwegian
hydropower is up to at least part of the task. Killingtveit led a
five-year, US $5.7 million research program on hydropower balancing,
which showed that existing hydropower reservoirs could "fairly
easily" move about 25 GW of energy in and out of storage without
damaging the environment-five times as much as they currently manage. The
key, he says, is installing pumps to shift water from one reservoir to a
higher one nearby, thus actively storing power rather than just deferring
production.
If
there is a limit to Norway's energy storage potential, it may ultimately
be the country's own grid. Statnett has begun a 10-year, $8 billion to
$10 billion grid upgrade, but it factors in only 3.5 GW of additional
power from the three cable projects. The question may be how many power
lines the Norwegians will accept to smooth Europe's departure from fossil
fuel power.
|
4) Solar
Power On The Rise
|
John Rogers, Fall 2014, Catalyst, Union of Concerned
Scientists,
http://www.ucsusa.org/clean_energy/our-energy-choices/renewable-energy/solar-power-technologies-and-policies.html#.VIedAst0w1I
Solar power generates
electricity with no global warming pollution, no fuel costs, and no risks
of fuel price spikes, and has the potential to help move the country
toward cleaner, reliable, and affordable sources of electricity.
Small-scale solar photovoltaic
(PV) systems, typically on rooftops, account for the majority of solar
installations, while large-scale PV systems and concentrating solar power
(CSP) systems constitute the majority of solar's overall
electricity-generating capacity.
All three are undergoing
rapid growth. Given the abundance of sunshine across the country, solar
power has the potential to supply a significant amount of electricity
that is both environmentally and economically attractive.

Their increasing
cost-effectiveness is largely a result of reductions in technology
prices, innovative financing, and growing networks of solar installers
and financial partners
Tax credits, rebates, and
other support in leading states can cut the total costs of a rooftop
system to under $10,000, though many solar customers are paying little or
nothing up front by utilizing solar leases or power purchase agreements,
which provide electricity from the system over a long period at
attractive fixed rates.
Costs for large-scale PV
projects have dropped more than household systems, to an average 60
percent lower than those for residential solar on a per-watt basis.
CSP systems have not
experienced the same cost reductions, but offer the important advantage of
being able to store the sun's energy as heat, and to use it to make
electricity when the sun is no longer shining.
Solar
power is viable throughout the United States
In a sunny location such
as Los Angeles or Phoenix, a five-kilowatt home rooftop PV system
produces an average of 7,000 to 8,000 kilowatt-hours per year, roughly
equivalent to the electricity use of a typical U.S. household.
In northern climates such
as in Portland, Maine, that same system would generate 85 percent of what
it would in Los Angeles, 95 percent of what it would in Miami, and six
percent more than it would in Houston.
For CSP, the best
resources are in the Southwest, though facilities have also appeared in
Florida and Hawaii.
·
- By early
2014, the United States had more than 480,000 solar systems
installed, adding up to 13,400 megawatts (MW)- enough to power some
2.4 million typical U.S. households.
- The U.S.
solar industry employed more than 140,000 people in 2013, a 53
percent increase over 2010, and is investing almost $15 billion in
the U.S. economy annually. There are currently more than 6,000
solar companies in the U.S., spread across all 50 states.
- Companies,
too, have embraced rooftop solar not only to improve their
environmental profiles but also to lower their operating costs.
- PV
systems require no water to make electricity, unlike coal, nuclear,
and other power plants. Likewise, solar panels also generate
electricity with no air or carbon pollution, solid waste or inputs
other than sunlight.
To
continue solar power's rapid growth, we must take steps to support its
continued acceleration
Important
focus areas include:
- Renewable
electricity standards. States should maintain and strengthen
their key policies for driving renewable energy investments,
including solar.
- Solar tax
credit. The federal investment tax credit that has been so
important for solar's rise is set to decline at the end of 2016 from
30 percent to 10 percent; Congress will need to take action to
sustain that support.
- Federal
power plant carbon standards. States should ensure that solar
plays a strong role in their plans to reduce emissions to comply
with the Environmental Protection Agency's new carbon standards.
- The full
value of solar. Assessing the full range of benefits and costs
of solar, particularly rooftop solar, will help policy makers decide
the most appropriate way to assist more people in adopting solar.
- Storage. Lower
costs and the greater availability of energy storage technologies
will help provide electricity more consistently and at times of peak
demand.
- New
utility business models. Utilities should modify their business
models to accommodate high levels of rooftop solar and encourage
continued solar development, from rooftops to large-scale projects.
- Research
and development. Solar's prospects will be enhanced by
continued progress in reducing costs-through greater economies of
scale, increasing cell and module efficiencies, improved inverters
and mounting systems, better heat transfer, and streamlined
transactions.
Back to table of contents
|
5) Water-Splitter Could Make Hydrogen
Fuel on Mars
|
Katharine Sanderson. New Scientist, 2014
http://www.newscientist.com/article/dn26204-watersplitter-could-make-hydrogen-fuel-on-mars.html#.VIn_U9LF_To
Making fuel on site for a
return trip to Mars may be a step closer. A cunning way to split water
into oxygen and hydrogen in two distinct steps could be a boon to both
astronauts and future Earthlings, enabling them to use renewable energy
sources for making hydrogen fuel.
Hydrogen fuel cells can power
vehicles ranging from cars to submarines and rockets. They can also heat
buildings, and double as portable power-packs for computers or other kit
used in the field. But existing methods for creating usable hydrogen gas
from water require a lot of electricity. That means renewable energy
sources like wind or sunlight, which are often patchy, are not reliable
enough.
It can also be hazardous
to scale up "artificial leaves", which make fuel from sunlight,
just like plants, says Lee Cronin at the University of
Glasgow, UK. This is because the low powers available don't produce the
gases quickly enough to keep them apart once they form. "All they do
is build up oxygen and hydrogen until they explode," he says.
Cronin and his colleagues
see this as a major obstacle to a future in which hydrogen fuel replaces
oil. To get around it, they built a device that uses a single pulse of
power to split water, so continuous energy is not needed.
Catch
and release
The device zaps water
with electricity to release oxygen, then a silicon-based chemical
mediator dissolved in the water mops up stray protons and electrons. When
it is full, the mediator turns blue, letting a human operator know it can
be removed and stored for later. When the hydrogen is needed, putting the
mediator in contact with a platinum catalyst allows those electrons and
protons to recombine to make hydrogen gas.
The whole process uses a
single whack of power, and patchy renewable energy will suffice for this,
says Cronin. In return, he says, 30 times as much hydrogen can be made
than from existing systems. The device could find uses generating power
in developing countries or for making fuel on Mars to power a rocket back
to Earth.
It is unclear whether
Cronin's device will be able to compete with other existing processes,
says Steve Reece, a water-splitting expert at Lockheed Martin in
Cambridge, Massachusetts. "It will be interesting to see how this
concept scales."
Journal reference: Science, DOI: 10.1126/science.1257443
Back to table of contents
|
|
|
Future Energy eNews is
provided as a public service from Integrity
Research Institute, a Non-Profit dedicated
to educating the public on eco-friendly emerging energy
technologies.
FREE copy
of the 30 minute DVD "Progress in Future Energy" is
available by sending an email with
"Free DVD" in subject and mailing address in body.
We need your generous support!
Please consider making a donation to our secure website
|
|
|